Editor’s Note: This is the first of a two-part series detailing the types of supplementary cementitious materials (SCMs) available and the role they play to enhance concrete strength.
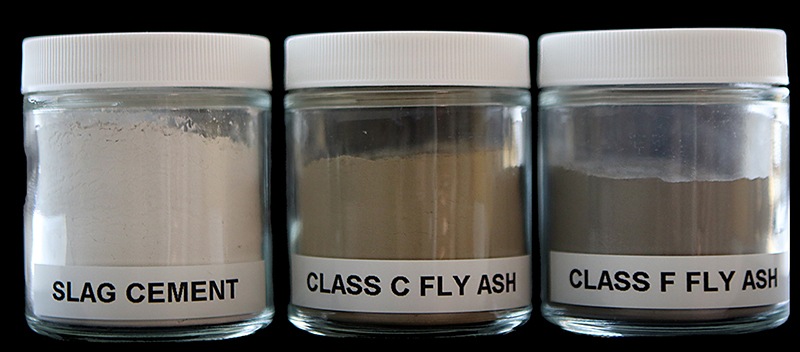
Cement and water have been known as a fantastic combination for millennia. They combine, react and create paste, which is a critical component in concrete. Paste serves as the binder that holds aggregates in place and gives concrete its engineering properties, making it a reliable and versatile construction material. But even this dynamic duo of cement and water are better off as a trio, particularly in terms of cement, water and supplementary cementitious materials.
What are supplementary cementitious materials?
Supplementary cementitious materials are natural materials or industrial byproducts that exhibit cementitious behaviors when combined with either water or water and other compounds. Many of these products if not used for other purposes typically end up in landfills or other disposal areas. SCMs enhance the results of ordinary portland cement (OPC) hydration reactions in concrete and are either incorporated into concrete mixes as a partial replacement for portland cement or blended into the cement during manufacturing. SCMs augment cement’s actions and can improve fresh concrete characteristics, strengthen hardened concrete properties and reduce raw material costs.
SCMs produced as a result of industrial manufacturing processes include fly ash, slag cement and silica fume. Natural SCMs, created by applying regimented heating and purifying processes to certain minerals, include calcined shale, calcined clay and metakaolin.
Hydraulic vs. pozzolanic
Cementing materials fall into two categories: hydraulic or pozzolanic.
Hydraulic is a description given to a material that chemically reacts with water. Portland cement is hydraulic. When portland cement and water mix, the reactions produce compounds that build upon one another and interconnect to create a matrix of hardened paste.
The main products of cement hydration reactions are calcium silicate hydrate and calcium hydroxide (abbreviated CSH and CH, respectively). CSH is largely responsible for building concrete’s most important engineering property – strength.
The term pozzolanic describes a material that displays little to no cementitious behavior when mixed with water alone. Alternatively, it chemically reacts and displays cementitious characteristics in the presence of water and other compounds. Pozzolanic substances act as cementing ingredients while consuming less-desirable material and contributing to the hardening cement paste matrix. The readiness and duration of the reaction over time is referred to as its pozzolanic activity. Similar to portland cement’s reactivity and behavior, pozzolanic activity is also based on the source of the material, its chemical makeup, the particle sizes and the material’s Blaine Fineness.1
Fly ash basics
Fly ash is a pozzolanic SCM and a byproduct of coal combustion. It is most commonly produced by coal-fired electric power plants. Depending on the makeup of the coal from which it originates, fly ash is categorized as either Class C or Class F, in accordance with ASTM C618.2 These categories are dictated by the amount of silicon dioxide, aluminum oxide and iron oxide in the ash, which influences the material’s behavior in concrete.
Fly ash particles are mostly solid and spherical, but some are hollow shells that contain smaller solid spheres of fly ash. Particles range in size from less than 1 micrometer to more than 100 micrometers. The average size of a fly ash particle is about 10 micrometers, or 1/10 the thickness of a standard piece of printer paper. Only a small portion of particles exceed 45 micrometers.
“The particles are very fine,” said Craig Wallace, technical director with Headwaters Resources. “The average size of a fly ash particle is about 1/10 the size of a particle of cement. By using fly ash you can increase the density of the cement paste. [The particles] fill the voids and increase the density of the paste, which makes it more impermeable.”
In the presence of water, these fly ash particles react with CH produced by cement hydration and replace it with the stronger, more desirable CSH. As cement hydration continues, more CSH and CH is produced. As long as the reactants are available in suitable quantities and other environmental factors are favorable, fly ash will continue to react with CH and create more CSH, adding strength to the concrete matrix.
Fly ash is used as a partial replacement for portland cement at a rate of 15% to 40% by mass of cementitious material for Class C ash, and 15% to 25% by mass of cementitious material for Class F ash. In some cases, fly ash can be used in excess of 40% by mass of cementitious content.
Fly ash generally lowers air content in a mix design, requiring a higher dosage of air-entraining admixture. How much air-entraining admixture will be needed is highly dependent on the fly ash’s carbon content, as well as its alkali content and fineness. According to Portland Cement Association’s Design and Control of Concrete Mixtures, “Increases in alkali contents decrease air-entraining admixture dosage requirements, while increases in the fineness and carbon content typically require an increase in dosage requirements. A Class F fly ash with high carbon content may increase the required admixture dosage by as much as 5 times compared to a portland cement concrete without fly ash. A Class C fly ash with low carbon content typically requires 20% to 30% more admixture.”
Good quality fly ash generally reduces water demand, similar to liquid chemical water reducers. The PCA document, “Optimizing the Use of Fly Ash in Concrete,” states: “Although the exact amount of water reduction varies widely with the nature of the fly ash and other parameters of the mix, a gross approximation is that each 10% of fly ash should allow a water reduction of at least 3%.”
It also states: “Coarser fly ashes or those with high levels of carbon generally produce a smaller reduction in water demand and some may even increase water demand.”
The implication is that decreased water demand can provide the same slump using less water, or can increase slump when using the same amount of water.
“It decreases water demand to obtain the same workable slump,” explains Wallace. “So if you get a 6-inch slump at 0.45 with cement, if you replace 25% of that cement with fly ash, you could obtain the same slump with about a 0.43 water to cementitious materials ratio.”
A lower water-cementitious material ratio contributes to increased strength, durability, abrasion resistance, freeze-thaw resistance and density, as well as decreased porosity and permeability.For a given slump, fly ash concrete can also exhibit improved workability compared with OPC. This also translates to an increase in ease of placing, consolidating and finishing practices.
Bleeding and segregation
Using SCMs will generally decrease bleeding. The finer the cementitious material and the higher the SCM content in the concrete mix, the lower the bleed rate and bleed capacity. Fly ash’s reduced water demand and increased fine particle content compared with OPC enables it to also decrease segregation. This makes fly ash beneficial for use in concrete made with aggregates having low levels of fines.
Heat of hydration
Class F fly ash decreases heat of hydration, and in some extreme cases contributes to heat of hydration only half as much as OPC. This makes it extremely useful in mass concrete applications or in hot-weather concreting, where it’s desirable to slow setting time and limit heat generation. Class C fly ash can either increase or decrease heat of hydration when compared with OPC concrete. This behavior is heavily dependent upon the ash’s calcium oxide content and other chemical makeup. The type and amount of compounds within it affect the reaction rates, which affect the speed at which strength-building compounds are produced and the rate at which heat is generated.
Effects on hardened concrete
Because fly ash affects heat of hydration and the rate at which cementitious reactions occur, the type of ash also affects setting time and early strength development.
“Precasters are somewhat limited in their ability to use fly ash because generally they like the concrete to cure in the forms for one day and strip the next. Sometimes fly ash doesn’t contribute to strength gain in the first 24 hours,” Wallace explains. “You have to have the first reaction with cement and water. The byproduct of that reaction is CH, then you have the secondary reaction with the fly ash. That usually occurs after the first 24 hours.”
Class F, having a lower heat of hydration, increases setting time and produces lower early strength results. Because Class C can have varying effects on reaction rates and heat of hydration, it has little to no effect on setting time and early strength gain. It is important to note that short-term strength results in concrete produced with fly ash should not be taken as an indication of long-term strength or quality. Fly ash typically retards the rate at which strength is developed early on – particularly up to three days after casting – but enables strength to increase at a steady rate long after OPC concrete’s strength development slows or tapers off.
Fly ash’s contribution to long-term strength development is marked but varies depending on its class. In some cases, particularly with Class C, concrete strengths exhibited between 1 and 28 days can be comparable to those of OPC concrete. In most cases, however, fly ash concrete’s increased long-term strength becomes evident beyond 28 days, sometimes nearing 90 days or more.
When deciding whether or not fly ash is right for a particular application, it’s important to not only consider the type of product in which the ash will be used, but also the environmental factors the product will face in service, as well as the ambient temperature and weather conditions to which the concrete will be exposed during placing, finishing and curing. Is fly ash required by the project specification? Is it disallowed for any reason?
“A lot of precasters are required to use fly ash in their products if they are doing work for DOT projects. It’s actually a requirement in a lot of states because it can make concrete more durable,” Wallace said. “Generally, it decreases bug holes. It also makes finishes smoother.”
As with any new mix design, it’s necessary to determine how each ingredient will interact with one another, including admixtures or other cement types. Setting time and strength development also need to be considered as well as production, stripping and shipping timelines. All SCMs have advantage and disadvantages in different scenarios, so it’s important to consider the variety of options and possible outcomes associated with each one.
Kayla Hanson, P.E. is a technical services engineer with NPCA.
Resources:
1. A material’s Blaine Fineness measures the total surface area of the sample particles per gram (cm2/gram).
2. Not all fly ash conforms to ASTM C618.
References:
Portland Cement Association, Design and Control of Concrete Mixes, 16th Edition
“Use of Fly Ash in Concrete.” ACI Materials Journal 84.5 (1987)