Many factors play a part to ensure the bond strength between reinforcement and concrete is strong
When you hear the word bond, you may think of 007 agent James Bond, who was made famous by Ian Fleming’s novels and corresponding hit movies. However, those who work in the concrete industry likely think of the interaction between concrete and reinforcement. Like the secret agent who never dies, concrete reinforcing bond ensures your precast products are long-lasting.
Concrete has excellent compressive strength, but lacks in tensile strength. Adding reinforcement creates a composite structure that can handle compressive and tensile stresses. But simply including the necessary reinforcement in the proper position doesn’t guarantee structural adequacy. The reinforcement must also adhere to the concrete in order to handle and resist compressive and tensile forces.
Steel bars and wire are traditional types of reinforcement that have excellent high tensile yield properties, ductility and moduli of elasticity that complement concrete’s attributes. To provide added durability when required, black steel bars are galvanized or epoxy-coated. Specialty stainless and new corrosion-resistant steels are also readily available. In addition, non-metallic reinforcing bar alternatives made from glass fiber reinforced polymers are available. With all these options, one key design property that must be understood is bond strength, a concept that has been thoroughly investigated and quantified through the efforts of academic research, the American Concrete Institute and ASTM International.1
Factors affecting bond
Reinforcing bond is developed by adhesion, friction and mechanical means.2 Adhesion offers the least amount of bond and is primarily created by the chemical interaction between the concrete paste and the reinforcement. Friction bond is the resistance created by the reinforcement and the concrete surface pressing against it. This is the predominant bonding mechanism for smooth bar reinforcement. Mechanical bond occurs when projections or deformations within the bar create a physical interlocking of the bar and concrete.
To properly place reinforcement, equations are used to calculate the minimum length of embedment for prohibiting slippage. This is called development length. Some of the critical variables are identical to those that affect bond strength, including bar size, concrete compressive and tensile strength, concrete cover, type of aggregate, bar spacing and coating (if used).
In addition, like development length, bond strength has a direct connection to the required length for reinforcement splices. Reinforcing splice design requirements, or lap lengths, have been developed through extensive bond research and testing.
To ensure the bond design assumptions are present and the corresponding development and splice lengths are adequate, precasters need to employ quality production practices for optimum reinforcing bond.
Concrete properties
Producing consistently high-quality concrete is important for compressive strength and durability and has a direct bearing on the reinforcement bond.
Research has shown concrete mixes that include hard aggregates like granites or basalts have better bond strengths than mixes with softer stone, such as limestone. Many lightweight aggregate concrete mixes have shown reduced bond strengths when compared with normal weight concrete.
In addition, concrete with a high water-cement ratio has a reduced bond capacity. High-slump mixes resulting from high water content can cause the concrete paste to settle away from the reinforcing steel and the void to fill with bleed water. This reduces all three bonding mechanisms. However, this has not proven to be a problem for higher-slump concrete produced by water-reducing admixtures. Still, precasters must be aware that if the self-consolidating concrete mix retards the expected strength gain, this would affect the rate of bond strength development.
When using concrete mixes other than SCC, proper consolidation practices are important to ensure optimum bond. Internal or external vibration will remove trapped air and associated voids. Good consolidation practices will also densify the mix around the reinforcement to improve both friction and mechanical bonding.
Reinforcement’s role
Proper reinforcement placement is critical for many reasons, including concrete bond. For example, if bar placement is less than the design cover, microcracks can lead to macrocracks and the resulting loss of bond. Steel corrosion and spalling may also occur.
During dry-cast production, correct reinforcement cage fabrication is important for optimum bond. If spacer clips are used to provide proper cover but the cage is not sized correctly and is forced into the formwork, it will be put into stress. Unlike wet-cast production, where concrete cures and hardens prior to form removal, the immediate form removal of the plastic dry-cast mix allows the stressed cage to spring back to its original position. This can result in a “slab off” of sections of concrete or create an excessive void around the steel, which greatly diminishes any anticipated friction bond.
Steel reinforcement
A few decades ago, any rust on steel reinforcement was considered deleterious and was removed. However, it’s now known that minor surface rust has no detrimental effect on the bond characteristics of reinforcing steel. In some cases, minor surface rust has improved friction bonding
characteristics. However, basic limits still apply. For example, rebar that shows scaling or pitting should not be used. Though steel reinforcement can be stored outdoors, best management practice dictates that steel should be placed on dunnage with no ground contact and stored in areas with no standing water.
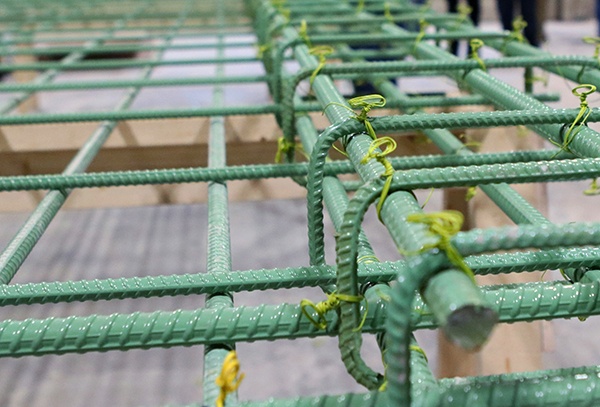
Corrosion-resistant reinforcement
In some instances, precast plants use corrosion-resistant steel reinforcement for concrete structures placed in harsh or extreme environments. Most corrosion-resistant alternatives are metallic-based or carbon steel with a protective epoxy coating, but glass fiber reinforced polymer bars are growing in demand. Before any corrosion-resistant reinforcement is included, however, the design characteristics must be carefully researched.
Epoxy-coated rebar is regularly used in precast structures; however, epoxy coating of deformed bar reduces friction and thus the bond strength with the concrete. Additionally, thicker coatings show less bond than thinner coatings, which could be attributed to the coating filling the bar deformation geometry and reducing the mechanical interlocking. Currently, design codes apply additional multiplication factors when calculating the development length when epoxy-coated reinforcement is used. Precasters must be aware of these differences and that a straight substitution of bare carbon steel with epoxy-coated rebar may not be appropriate in some cases without some development length adjustments.
Types of corrosion-resistant metallic rebar include stainless steel, low-carbon, high-strength chromium steel products, zinc-coated galvanized bar and dual-coated rebar. All these products are undergoing or have undergone testing regarding bond strength per ASTM A944. Results vary, but in general the bond strength of corrosion-resistant materials is within 95% of the accepted values for traditional carbon rebar. When using CR reinforcement, precasters need to carefully review the production drawings to determine if any unique development length or splice overlaps are required. Also, precasters need to investigate if a stock substitution of CR reinforcement for standard carbon steel rebar can be done.
Fiber reinforced polymer bars have many beneficial attributes – primarily, high tensile strengths, corrosion resistance and the ability to be used in environments sensitive to electric currents. However, there are also many other material attributes that need to be addressed prior to general design acceptance. One issue under investigation is appropriate bond strength, which has proven difficult to determine due to the various types of FRP available, and the fact that its surface is softer than steel.3
If you cast a structure using FRP bars, pay close attention to the manufacturer’s recommendations and fabrication details. If permitted to substitute one FRP manufacturer’s bar with another, ensure they are equal to the original design expectation.
Fiber reinforcement
Although the actual steel fibers in fiber-reinforced concrete are small, bond strength and development length are still important. To achieve bond strength, there are many proprietary configurations of special hooks or helical shapes, surface roughness or chemical bonding.
According to Yaun and Graybeal (2015), “The discrete steel fiber reinforcement included in UHPC allows the concrete to maintain tensile capacity beyond cracking of the cementitious matrix. The combination of the matrix and fiber performance allow for a reduction on the development length of reinforcing steel bar, thus providing the potential for a redesign of some structural systems such as field-cast connections between prefabricated bridge elements.
“It was found that the bond behavior of deformed reinforcing steel in UHPC is different from that in traditional concrete in many aspects. In general, the reinforcing steel development length in UHPC can be significantly reduced.”4
Fibers used in conjunction with UHPC can greatly increase bond strength, which results in reduced development length requirements and reduced reinforcement splicing requirements, leading to more structural precast bridge component connections.
Form oil
Like rust, the question of how much detrimental effect form oil has on reinforcing bars is now the subject of research.
The current code provisions within ACI 301, “Specifications for Structural Concrete,” section 2.3.1.15, state, “Do not allow formwork release agent to contact reinforcement.” The NPCA Quality Control Manual for Precast Concrete Plants, section 4.3.2, also states, “Reinforcement and other items to be embedded in concrete shall be free of form release agent.”
However, recent research5 casts doubt on this intuitive school of thought and current code language. Until additional data verifies results, form oil should be removed from reinforcing elements, particularly on epoxy-coated and smooth FRP bars.
Agent 007 reinforcement
Significant research and engineering goes into the design and production of a strong, durable, safe and resilient precast concrete structure. The next time you are at the movies watching the latest 007 thriller, know there is another “bond” working to keep you safe.
Eric Carleton, P.E., is NPCA’s director of codes and standards. He is also an ASTM Award Merit recipient and currently serves as vice-chairman of ASTM Committee C13 on Concrete Pipe.
Resources:
1 ASTM A944, “Standard Test Method for Comparing Bond Strength of Steel Reinforcing Bars to Concrete Using Beam-End Specimens,” and ACI 408R-03, “Bond and Development of Straight Reinforcing Bars in Tension,” provide better correlation and comparison of research results internationally. This practice is important for new reinforcing products.
2 Zuo and Darwin (1998), “Bond Strength of High Relative Rib Area Reinforcing Bars,” Structural Engineering and Engineering Materials SM Report, No. 46.
3 Bond of FRP Reinforcement in Concrete – A Challenge, Article in Mechanics of Composite Materials, 39(4):315-328, July 2003.
4 Bond of Reinforcement in Ultra-High-Performance Concrete by Jiqiu Yuan and Benjamin Graybeal. ACI Structural Journal, V. 112, No. 6, November-December 2015.
5 Belarbi, A., Richardson, N.D., Swenty, M.K. and Taber, L.H., (2010), Effect of Combination on Reinforcing Bar-Concrete Bond, Journal of Performance of Constructed Facilities, ASCE, Vol. 24, No. 3, May-June.